For the first time, ultrasound imaging has been used to image a patient's brain outside a hospital setting while the subject performs tasks such as video gaming and guitar playing. Using a cranial prosthetic that provides a literal window onto the brain through which ultrasound can pass unhindered, the Caltech-led proof-of-concept study opens the door to a better understanding of the brain, improved diagnostics for brain conditions, and the possibility of a direct interface between the brain and a computer that could allow patients to, for example, control a cursor on a computer screen with their thoughts and intentions alone.
The study is described in a paper appearing in the May 29 issue of Science Translational Medicine.
The hurdles impeding a deep understanding of the brain, as well as its direct interaction with external devices such as robotic arms and computers—are incredibly high. The brain, by any measure, is an incredibly compressed piece of machinery. Because it accomplishes so many tasks with the cubic centimeters allotted to it, precision instruments are required to achieve the sensitivity and resolution necessary to see what the brain is doing. Techniques exist for doing so in small areas of the brain in animal models. However, managing this feat on a larger scale is challenging due mainly to the human brain's bony casing, the skull.
For eight years, Mikhail Shapiro, the Max Delbruck Professor of Chemical Engineering and Medical Engineering, and a Howard Hughes Medical Institute Investigator, has been collaborating with Richard Andersen, the James G. Boswell Professor of Neuroscience and director and leadership chair of the T&C Chen Brain–Machine Interface Center, and researchers at Inserm in Paris, to pioneer the use of functional ultrasound imaging as a brain–computer interface.
Diagnostic ultrasound works a bit like the echolocation used by dolphins or bats. High-frequency sounds are emitted and bounce off boundaries between the materials they encounter, including soft tissue and blood. Taken a step further, a technique called functional ultrasound imaging uses high frame rate imaging and computer algorithms to image blood-volume changes in the brain. When the brain's neurons are active, they use metabolic resources that are supplied via the blood stream. By measuring changes in blood volume, the researchers can infer when and where the brain's activity is changing.
In 2021 and 2023, Andersen and Shapiro's teams published research conducted on nonhuman primates that showed that data from an ultrasound of the brain could successfully be decoded to indicate an intention formed in the brain, even before the action corresponding to that intention can be carried out.
Now they have demonstrated that this can also be achieved in an adult human in a natural setting, i.e., outside the operating room.
To produce these precision ultrasound readings of brain activity, a portion of the skull must be removed and replaced with a "window" through which ultrasound signals can pass. Volunteers for such a procedure are unsurprisingly scarce, but as it happens, numerous people must have a portion of their skull removed—what's known as a craniectomy—to relieve pressure after a severe traumatic brain injury.
"A traumatic brain injury causes the brain to swell. If we remove a portion of the skull and allow the brain to expand and then gradually return to its normal size, we greatly improve outcomes for patients," explains Whitney Griggs (PhD '23), a medical student in the UCLA-Caltech Medical Scientist Training Program, and a co-author of the new paper.
Eventually, the brain needs its protective covering replaced. "If the bone is not too damaged from the injury itself, it can be replaced," Griggs says. "But, often, this is not possible, and neurosurgeons must use other materials, such as titanium mesh or custom-built cranial implants."
Claire Rabut, formerly a postdoc in Shapiro's lab and currently a visitor in chemical engineering at Caltech, experimented with materials used for cranial implants and found that polymethyl acrylate, already favored for its sterility and strength, was also transparent to the acoustic signals relied upon by ultrasound. Sumner Norman, also a former postdoc in Andersen's and Shapiro's labs, worked with Rabut to create an implant that, according to Norman, "would serve not only as a safe and cosmetically transparent prosthetic, but also be transparent enough that ultrasound waves could achieve the high sensitivity needed for functional brain imaging."
The resulting implant was 4 millimeters thick overall but had a 2 millimeter-thick window through which an ultrasound transducer could image a brain region known as the posterior parietal cortex and, behind it, the motor cortex. "This area of the brain, which is important for forming intentions and carrying out motor actions, has already been thoroughly studied via other methods in our lab," Andersen says. "With ultrasound, we can image the brain up to a resolution of one-fifth of a millimeter."
The team worked with Charles Liu, a neurosurgeon at USC and visiting associate in biology and biological engineering at Caltech, to identify patients whose brain injuries would require a cranial implant and for whom ultrasound imaging would not create any additional risk. After recovering from surgery, the selected patient was asked to perform a few simple tasks while ultrasound scans were taken of their brain. "Just as had been the case with nonhuman primates, the patient's ultrasound data indicated intentions—move this joystick, strum this guitar—while the actions themselves were taken," Liu says.
Ultrasound is not the first technology for imaging the brain, but it has several advantages over other imaging methods such as functional magnetic resonance imaging. "If you've ever had an MRI, you know that it was big and loud, and you had to lie very still inside a tube as the scan was done," Rabut says. "Ultrasound, by contrast, is portable and relatively small. You have a handheld probe that may cost a few thousand dollars as opposed to the million dollars needed to purchase an MRI machine. More importantly, for research purposes, you cannot observe the brain activity of a patient who is sitting up, awake, and doing very active things with MRI. With ultrasound, you can study brain activity in normal, real-life settings."
Replacing a portion of a person's skull is less invasive than some other techniques used to monitor brain activity. "The surgery to place a cranial implant is done on top of the dura, the sturdy envelope of tissue that protects the brain," Rabut explains. "There is very little risk of infection. In contrast, if you implant electrodes in the brain, you are performing surgery on the brain itself. Not only that, these electrodes can only monitor a few neurons. With ultrasound, we can image a much wider field." (However, microelectrode arrays have higher temporal resolution and can record from populations of single neurons, so both technologies have their pros and cons for brain–machine interfaces.)
"There are a number of interventions that require removal of a portion of the skull," Shapiro says. "So, there are many patients who could potentially benefit from a cranial implant that is transparent to the acoustic signals that ultrasound uses."
The paper is titled "Functional ultrasound imaging of adult human neural activity through an acoustically transparent cranial prosthetic." Co-authors include Rabut, Norman, Griggs, Andersen, and Shapiro of Caltech; Liu and Jonathan Russin of USC; and Vasileios Christopoulos of UC Riverside and a visiting associate in biology and biological engineering at Caltech. This work was supported by the National Institutes of Health, the T&C Chen Brain–Machine Interface Center, the Boswell Foundation, the National Eye Institute, a Josephine de Karman fellowship, the UCLA–Caltech Medical Scientist Training Program, a Della Martin postdoctoral fellowship, a Human Frontier Science Program Cross-Disciplinary Fellowship, the Howard Hughes Medical Institute, and the USC Neurorestoration Center.
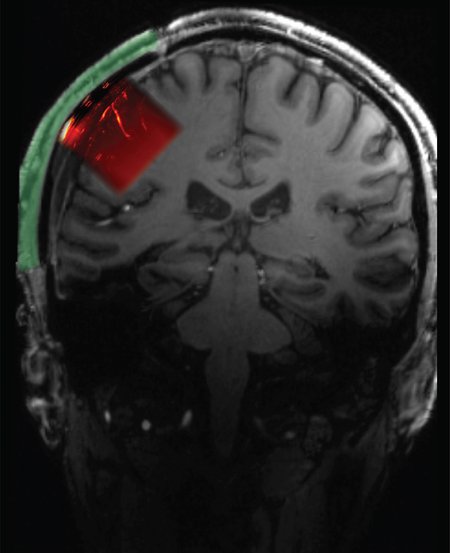
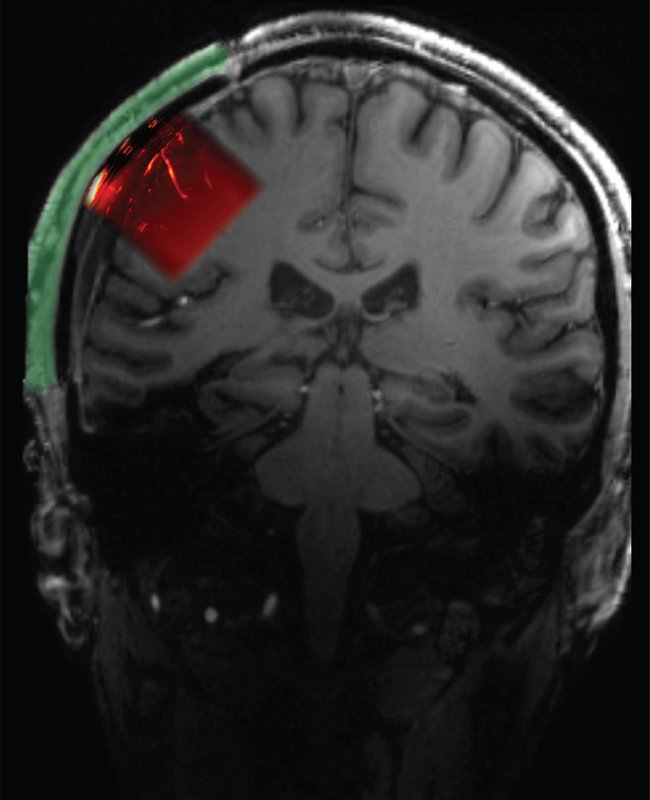